PITTING CORROSION
UNIFORM CORROSION
Pitting corrosion is a form of extremely localized corrosion attack. It occurs in metal alloys that form a protective thin surface oxide film such as stainless steels (SS) and alloys of aluminum, zinc, nickel, titanium, and magnesium. The corrosion rate inside pits can be several orders of magnitude greater than that typically found during uniform corrosion of these alloys. Pitting attack will lead to metal perforation, often found in metal pipe applications exposed to a mild or aggressive corrosion media. This type of corrosion attack occurs in the presence of chloride, bromide, iodide, fluoride, or sulfates. Pipes used in industrial applications fail typically by perforation caused during localized pitting attack.
Localized pitting attack in non-chloride aqueous environments can happen from microbial corrosion. This process creates a microenvironment distinct than that of the bulk environment in pH, dissolved oxygen, and the presence of organic and inorganic species. Alloys of copper, aluminum, and nickel, as well as steels, are susceptible to corrosion and pitting attack by microbial corrosion. Copper pipes and tubings used for water lines are known to develop pinhole leaks attributed to pitting from microbial induced corrosion (MIC).
Pitting corrosion is evaluated according to ASTM G46 and it is measured by a cyclic potentiodynamic polarization test according to ASTM G61 for iron, nickel, and cobalt-based alloys. During the cyclic polarization sweep, pitting is detected when a sudden rapid and continuous current rise is observed once the metal’s pitting potential is reached. The pitting potential defines the onset of stable pit growth. Some alloys can be ranked based on their pitting potential value. The more anodic the pitting potential of an alloy is, the more resistant to pitting attack the alloy is. The graph below compares the polarization behaviors of 303 and 304 stainless steel in an aqueous solution containing 3.5% NaCl, simulating the chloride content in a seawater environment. The graph shows that 304 SS has better pitting corrosion resistance than 303 SS. Pitting can also be determined by a potentiostatic technique measuring current values as a function of time for different applied constant anodic potentials. In this case, pitting will develop at the lowest applied constant potential causing a sudden continuous current rise due to stable pit growth. An example is shown in the adjacent graph for 304 SS in NaCl-saturated aqueous solution polarized at 0.300 V.
There is a critical pitting temperature, CPT, at which metals start to pit and is determined according to ASTM Standard G48 method C for nickel-base or chromium-bearing alloys, and method E for stainless steels. The potentiostatic technique to determine CPT is done according to ASTM G150 for stainless steels and related alloys.
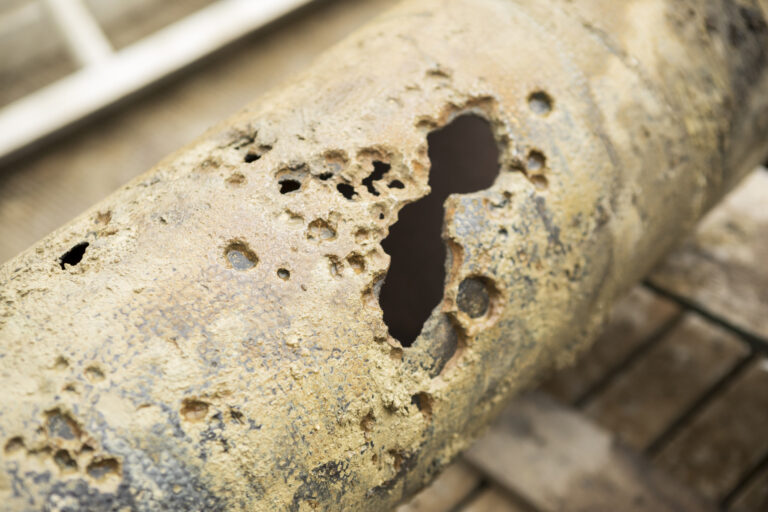
Localized corrosion in an industrial pipe caused by pitting corrosion attack.
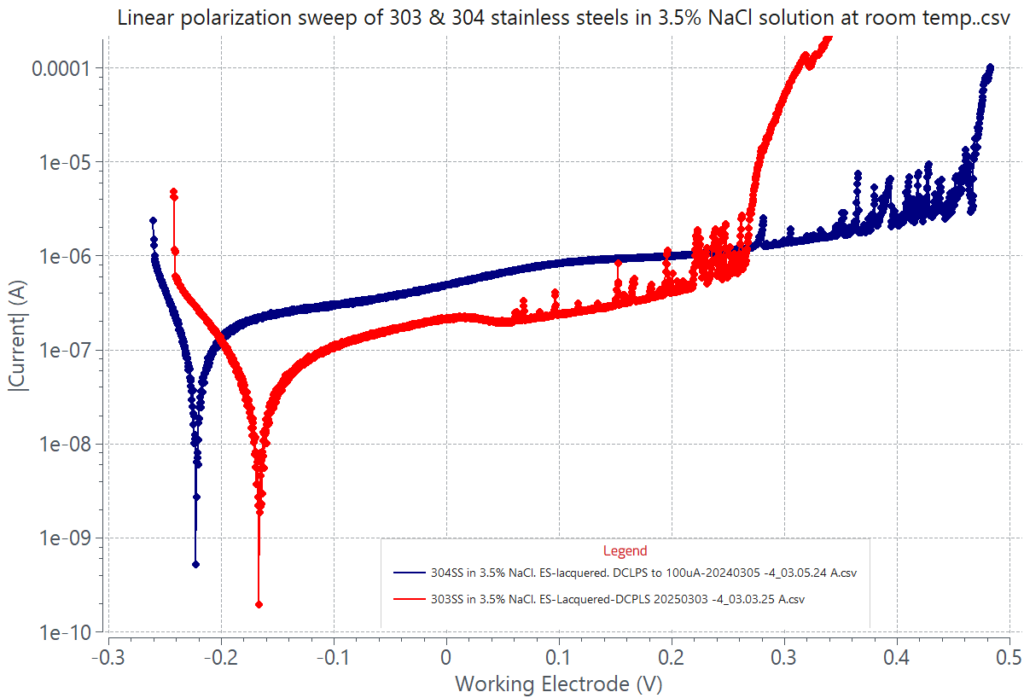
DC potential polarization sweep of 1 cm2 area 303 (red graph) and 2 cm2 area 304 (blue graph) stainless steel in 3.5% NaCl-aqueous solution. Both alloys exhibit pitting attack and fast metal dissolution revealed by the rapid and continuous rise in current at about 0.265 V for 303 SS and at about 0.460 V for 304 SS; relative to a Ag/AgCl reference electrode. The region in the graph with small intermittent sharp rises in current defines a metastable pitting stage at which newly formed pits quickly re-passivate. Continuous pit growth occurs at and above the pitting potential.
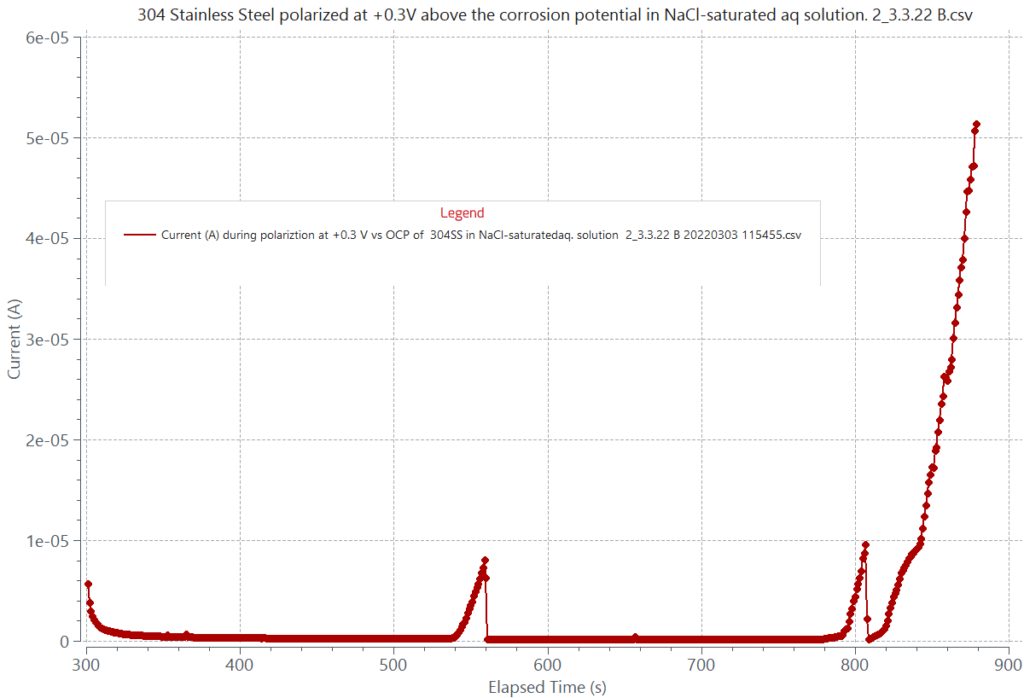